Vaccines and Treatments for COVID-19
This is the second of my posts, in which I cover the primary medications and vaccines undergoing testing for COVID-19. For an introduction to the science of COVID-19, see this page. This post is not exhaustive because there are numerous candidates in early development, but I describe how vaccines and the immune system work and potential safety issues around vaccines and treatments.
Current Treatments for Viral Illnesses
As a viral illness with no known cure, COVID-19 is treated with supportive care, which includes fluids, fever reducers, artificial nutrition, and in severe cases, oxygen 1. Oxygen is typically delivered through a ventilator -- a tube is inserted through the nose into the trachea, and the machine physically pushes air into the person’s lungs to simulate breathing. This is used for patients with difficulty breathing but whose lungs are still functioning. In the most severe cases with multi-organ failure, an extracorporeal membrane oxygenation (ECMO) machine removes a person’s blood, oxygenates it, and then returns it to the patient’s body. Supportive care targets the symptoms, not the cause, of COVID-19.
Medications in Clinical Trials
Several treatments for COVID-19 are already being tested in clinical trials. These were previously approved by various countries to treat other conditions, so the development of such repurposed drugs will be faster than that of new compounds. An important distinction to make is between tests performed in vitro (in cells grown in culture dishes) and in vivo (in animals or humans). The preclinical stage consists of in vitro tests and in vivo animal studies, followed by clinical trials in humans. Drugs and vaccines sometimes behave differently in vitro and in vivo.
One drug is Remdesivir, an antiviral that was originally studied by Gilead Sciences for Ebola. Antivirals do a variety of things, but their goal is to inhibit viral infection, production, or release without interfering with the normal host cellular activity. Coronaviruses, which have RNA genomes, use RNA-dependent RNA polymerases (RdRPs) to copy their genome. Human cells transcribe mRNA from DNA and copy our own DNA in the nucleus, but they seldom copy RNA from RNA. DNA and RNA are made up of molecules called nitrogenous bases, and remdesivir is similar to one one of these bases. It has been chemically modified to prevent more bases from being attached to it, so remdesivir is incorporated into new viral RNA as it is replicated and halts the process.
Another treatment under consideration is hydroxychloroquine (HCQ), which may have multiple therapeutic effects for COVID-19. HCQ may raise the pH of endosomes, potentially inhibiting one of the proteases that SARS-CoV-2 uses to infect cells 2. HCQ also has anti-inflammatory effects, so many people take HCQ for autoimmune diseases, such as lupus and rheumatoid arthritis. A hyper-inflammatory response is responsible for much of the lung damage induced by SARS-CoV-2 infection, so HCQ could reduce disease severity in this way. The University of Pennsylvania began a trial in early April 2020 testing HCQ in three groups of people -- COVID-19 patients quarantined at home, hospitalized COVID-19 patients, and non-infected healthcare workers taking HCQ as a preventive measure -- with control groups 3.
TMPRSS2 is a protease in cell membranes that may be involved in SARS-CoV-2 entry into cells. Camostat mesylate is a clinically-proven TMPRSS2 inhibitor licensed in Japan and South Korea to treat pancreatic inflammation. It was shown to inhibit SARS-CoV-2 infection of lung cells in vitro and SARS-CoV infection in mice 4, and a trial is underway in Denmark 5.
The antiviral combination Lopinavir-Ritonavir that was approved by the FDA in 2000 against HIV 6. When SARS-CoV-2 viruses are replicated in host cells, polypeptides -- sequences of amino acids -- are made from the viral genome and then cleaved by a viral protease to make functional proteins for new SARS-CoV-2 viruses. These antivirals are protease inhibitors, so they inhibit the viral protease and prevent assembly of new viruses. A trial with 1220 participants began at the end of March 2020 in Toronto, Canada 7.
None of the aforementioned treatments has been fully tested in clinical trials against SARS-CoV-2, and although there may be anecdotal evidence of their efficacy, there are numerous confounding variables. In small studies without proper control groups, we can not distinguish between therapeutic effects and the immune system clearing an infection on its own. Off-label drug use is very risky because many drugs have been approved only for use in certain groups of the population, and the doses required for antiviral activity may be different from those that have already been tested. In short, you can only make conclusions about how a drug works and its side effects in humans if you have tested it in the exact way you intend to use it on the target population in randomized clinical trials.
Convalescent Plasma and other Antibody Therapies
Convalescent patients are those who are recovering (or recovered) from a disease, and plasma contains antibodies and other proteins. Plasma from patients who recovered from COVID-19 may be able to treat current patients. For all 10 patients with severe COVID-19 who were given convalescent plasma in Wuhan, China, SARS-CoV-2 RNA level dropped, and blood oxygen, lymphocytes (B cells, T cells, and natural killer cells), and neutralizing antibodies increased. None of the patients died. However, there were some confounding variables because the patients were given other treatments at the same time, such as antivirals (including remdesivir), antifungals, antibiotics, and glucocorticoids, if needed 8. This was not a clinical trial, and other treatments were given in an effort to improve the patients’ conditions.
Convalescent plasma is a form of passive immunity because antibodies are acquired from a source other than a person’s own immune system. Another example of passive immunity is antibody transfer from a mother to a fetus through the placenta. There is evidence that these maternal antibodies can inhibit production of the baby’s own antibodies, so infants under 6 months are not given vaccines to give time for the maternal antibodies to be naturally degraded. Similarly, convalescent plasma recipients may not be able to mount their own immune responses if the administered antibodies work very well. Knowledge of the structure of the SARS-CoV-2 spike protein can be used to design monoclonal antibodies to stop it from binding to ACE2 and infecting cells.
Tocilizumab is a monoclonal antibody that binds to receptors for an inflammatory cytokine called interleukin-6 (IL-6). It is used to treat rheumatoid arthritis because elevated IL-6 has been associated with the disease. There is some evidence that severe COVID-19 patients may also have elevated IL-6 9, so two trials, each with 300-400 participants with severe COVID-19, are underway to assess the safety and efficacy of tocilizumab 10,11. The following image shows the six major therapeutic routes being tested in clinical trials.
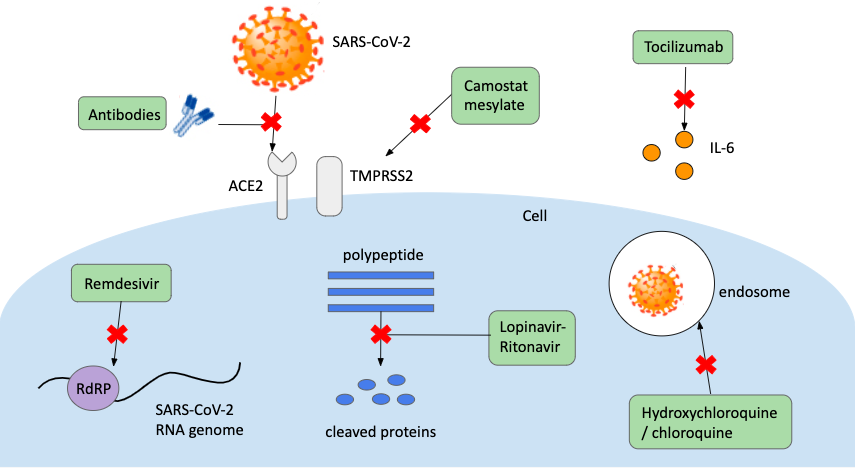
Disclaimer on Antibodies
Antibodies that are successful at blocking infection are called neutralizing antibodies. There are also numerous non-neutralizing antibodies that do not prevent infection, but many of which bind still bind to pathogens. Immune responses are often measured by the level of specific antibodies in a binding assay, but some of these antibodies could be non-neutralizing and therefore not protective. Moreover, non-neutralizing antibodies can sometimes compete with neutralizing antibodies or facilitate viral infection, which is discussed later.
A Primer on the Human Immune System
The immune system is separated into two main arms -- innate and adaptive. The innate immune system broadly attacks all antigens (molecules that cause an immune response). Physical defense mechanisms like skin and hair first attempt to stop pathogens (disease-causing microbes) from entering the body. But pathogens can enter through open wounds, or the gastrointestinal, respiratory, and genitourinary tracts because these open to the outside world, so these tissues are lined with protective epithelial cells. These cells secrete mucus, stomach acid, and saliva that create hostile environments for pathogens or contain antibodies to neutralize viruses and toxins and prevent pathogens from getting to epithelial cells 12. In the blood, cytokines induce inflammation and immune cells engulf pathogens, and molecules called interferons interfere with viral replication. After a week or so, if the infection has not been cleared, the adaptive immune system kicks in.
The adaptive immune response is specific to each pathogen. Naive cells are immune cells that have not yet encountered an antigen, which is a piece of a pathogen that causes an immune response. The first time the immune system encounters a pathogen, T cells kill infected host cells and activate the immune system, and B cells secrete antibodies specific to the antigen. Upon activation, memory B cells and T cells are also produced. Memory cells are longer-lived than other B and T cells, and they initiate an immune response in the future if the same antigen is encountered again, and this response will be faster than the first. Antibodies will bind to a pathogen with greater affinity during subsequent exposures in a process called affinity maturation 13, so many vaccines are given in multiple doses to get high affinity memory B cells. Immunity wanes over time because populations of memory cells that are not used frequently drop, which is why booster vaccines, such as Tdap (tetanus, diphtheria, and pertussis), are given throughout your life to “remind” your immune system of these pathogens.
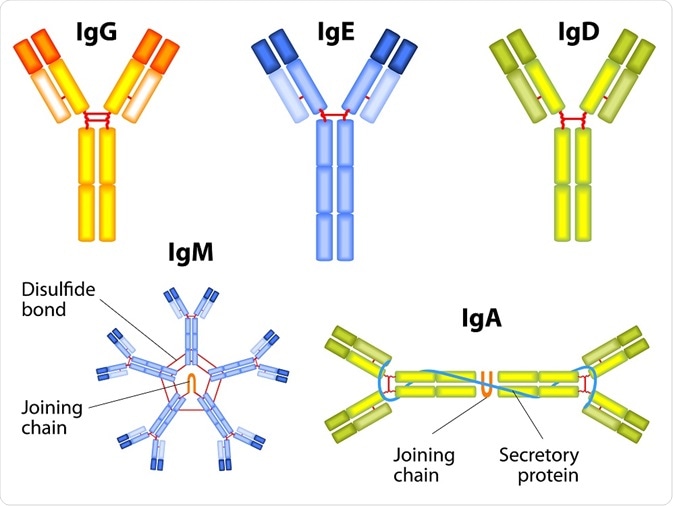
IgGs are primarily responsible for immunological memory and are of particular significance in the adaptive immune response. The graph below is a typical concentration profile for IgGs. The rate of IgG production is faster after the second antigen exposure, and more IgGs are produced. The graph also shows IgM, which activates B cells and components of the innate immune system, but it does not contribute to immunological memory, since its secondary response is neither faster nor stronger than its primary response.
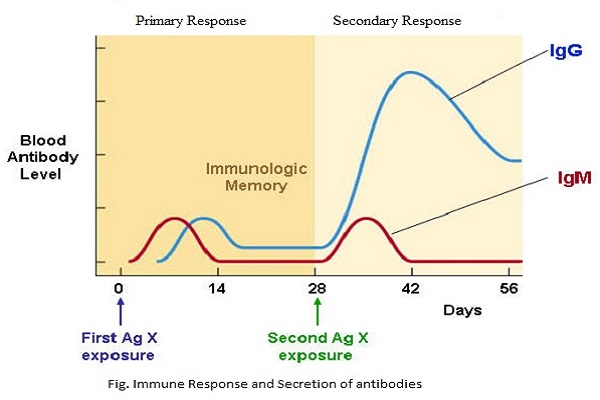
What are Vaccines?
Vaccines are any molecules administered prophylactically to individuals to prepare the adaptive immune systems for future infections. There are several major types of vaccines -- whole pathogen, subunit, and nucleic acid. A whole pathogen can be live-attenuated by modifying the virus so that it doesn’t cause disease, or inactivated by treating it with heat, radiation, or chemicals, leaving just the protein coat and surface proteins behind. Whole-pathogen vaccines typically elicit the strongest immune responses and provide longest lasting immunity, but all pathogens can not be made in an inactivated, but still immunogenic, form. (Immunogenic means eliciting a strong immune response).
Subunit vaccines contain only a small part of a pathogen, so they’re safer than whole pathogens, but an adjuvant may need to be added to make it more immunogenic. Nucleic acid vaccines consist of DNA or RNA encoding a protein from a pathogen. When a nucleic acid vaccine is injected into a patient, the patient’s cells make viral proteins from the nucleic acid, and the immune system reacts to them because the proteins are recognized as non-self (foreign). Nucleic acid vaccines are easier to produce than the others because large quantities of protein do not have to be made and purified first. They are also safer because they do not expose manufacturers or patients to a potentially infectious particle. 14
DNA is more stable than mRNA, but to produce viral proteins, DNA must get to the nucleus of a cell, where it risks altering the genome (a cell's DNA is housed in the nucleus). Moreover, studies have found that DNA was less efficient at entering cells in the first place 15. The weaknesses of mRNA, on the other hand, can be remedied: modifications have been made to mRNA to make it more stable and less likely to elicit an immune response against itself 16.
Nucleic acid vaccines are typically delivered in nanoparticles. Nanoparticles are often used as vectors to safely and reliably deliver a therapeutic agent, such as a medication or nucleic acid. They can be coated with molecules that localize them to a certain tissue (targeted drug delivery) or with viral proteins to mimic the virus itself and stimulate an immune response. Nanoparticles also prevent the nucleic acid from being degraded before it gets to cells.
Why do Vaccines Take So Long to Develop?
Any treatment that is administered to the population must first be thoroughly tested for its safety and efficacy. Vaccines prepare the immune system for an infection by exposing it to a small piece or weakened version of a pathogen. In the preclinical stage, a vaccine candidate is identified and tested in animal models (sometimes responses are very different in mice and non-human primates, which complicates testing). Safety testing for vaccines is critical because they are given to healthy people and must work in people of all ages and health conditions.
The clinical development stage consists of 3, sometimes 4, phases with progressively larger groups of subjects. Each phase can take a year or more to allow adequate time for the adaptive immune response to reach its height and to monitor side effects and adverse reactions, which may not occur immediately. Later phases may be longer to study the need for multi-dose vaccines and how immunity changes over time. Vaccine efficacy is assessed in all phases, typically by measuring markers like antibodies, immune cells, and cytokines. 17
Under normal circumstances, vaccine clinical trials typically take several years. The 18 month estimate 18 given by Dr. Anthony Fauci, director of the National Institute for Allergies and Infectious Diseases (NIAID), is extremely fast by all trial standards. Most scientists are skeptical that a vaccine will be ready in this short time because any problems, such as side effects, poor immune responses, or manufacturing issues, will delay development. Although a vaccine candidate may be identified in only a few months, passing all the safety inspections takes much longer. As described earlier, the adaptive immune response takes a few weeks to reach its height and settle down. Only after this, chemical markers of memory cells can be measured. Multi-dose vaccines, given over longer periods of time, should also be explored because they can enhance immunity through repeated exposures.

There is concern about a phenomenon called antibody-dependent enhancement (ADE) in coronaviruses. This occurs when antibodies promote future infection, rather than inhibit it. Typically, non-neutralizing or poorly neutralizing antibodies bind to Fc receptors on certain immune cells and help the virus infect them 19. However, the mechanism of infection through ADE is not well understood.
Some non-neutralizing antibodies promoted SARS-CoV infection in vitro. Even though these antibodies bind to the virus, their non-neutralizing ability is actually harmful 20,21,22. Treatment with convalescent plasma could be problematic because plasma contains polyclonal antibodies, some of which could be non-neutralizing. Broadly neutralizing antibodies may have better therapeutic effects on COVID-19 patients. There is a lot of research focused on developing rapid tests to find broadly neutralizing antibodies in convalescent plasma 23. Structural studies of the SARS-CoV-2 spike protein are also important for designing neutralizing monoclonal antibodies to block the spike protein from binding to ACE2.
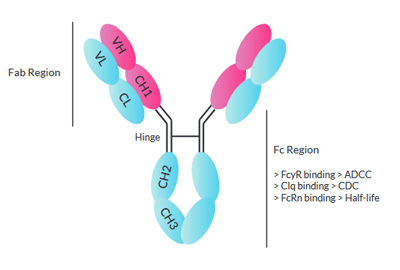
Despite reports of patients in China testing positive for SARS-CoV-2 after they had been discharged once, it could have been due to false negatives or low viral load in the middle of an infection. A study of rhesus macaques infected with SARS-CoV-2 twice demonstrated that the monkeys did not develop COVID-19 symptoms or detectable viral RNA after the second infection 24. Additionally, several studies on SARS-CoV in the early 2000s showed that potent neutralizing antibodies can be elicited in animals that protected them from future infections 25,26,27. However, the SARS outbreak ended before trials in humans could begin. ADE or other adverse effects associated with antibodies may or may not pose a problem for SARS-CoV-2 vaccination efforts, but it is difficult to know without studies in animals and humans.
SARS-CoV-2 Vaccines in Development
The World Health Organization (WHO)’s website shows all vaccine candidates being tested in preclinical and clinical trials under “Draft Landscape of COVID-19 Candidate Vaccines.” It is beyond the scope of this post to describe all of the preclinical vaccine candidates, but I will address the two that are in phase I clinical trials.
One, underway in Seattle, is an mRNA vaccine developed by Moderna and the NIAID Vaccine Research Center known as mRNA-1273 28. A sample of 45 healthy adults have been randomly assigned to receive one of three vaccine doses and will be followed for a year. The goals of the study are to make sure that the vaccine is safe and to study participants’ immune response by measuring IgGs in a binding assay against the SARS-CoV-2 spike protein 29. This vaccine consists of mRNA enclosed by a lipid nanoparticle.
The other vaccine candidate, which is also a nucleic acid vaccine in a viral vector, was developed by CanSino Biologics Inc. in Tianjin, China 30,31. This vaccine is an inactivated adenovirus-5 containing the gene (DNA) for the SARS-CoV-2 spike protein. The design was modified from an adenovirus-5 vector containing the gene for an Ebola virus surface glycoprotein, developed in 2015. In a phase I trial for Ebola, this vaccine did not cause severe adverse events and produced antibodies in all participants 32.
My last point is that a vaccine is unlikely to provide lifelong immunity. None of the respiratory viruses, such as influenza, respiratory syncytial virus, coronaviruses, adenoviruses, and rhinoviruses, provides lifelong immunity after infection. Immunity to cold-causing coronaviruses lasts for a year or so, and some SARS-CoV patients had antibodies for approximately 2 years 33, but humans are repeatedly infected with respiratory viruses throughout their lives. This is not the case for diseases like chickenpox, measles, mumps, rubella, and tetanus, which do provide lifelong immunity through infection or vaccination (but may require boosters). We don't know the reason for this because both slowly and rapidly mutating viruses do not provide lifelong immunity. We may have to cope with endemic SARS-CoV-2 in the future because its transmissibility makes it difficult to eradicate, but there is good reason to hope for an effective vaccine. Furthermore, it is likely that immunity will provide at least some protection against severe disease in the future (so you would get a mild or even asymptomatic case if you're reinfected with SARS-CoV-2), though we do not know the details of immunity.
Leave a Comment